Scientists have developed a multi-physics thermal model for panels with a natural convection cooling loop and tested it against experimental data. They have also experimented with varying the coolant channel thickness and different coolants. The highest measured electrical efficiency reached 15.45%.
An international research team, led by the University of Manchester, studied the thermal modeling of floating photovoltaic (FPV) panels with a natural convection cooling loop (NCCL). The researchers created a novel 2D multi-physics numerical model to evaluate the system using varying coolant channel thicknesses and nanofluids.
“The NCCL is a passive cooling system which removes heat from the PV panel and transfers it to a large body of water, such as a lake, river, or a dam,” the group explained. “It consists of three main elements: the heat sink section at the base of the loop, immersed in the water body; the PV module, with a length of 0.75 m, above the heat sink section, which receives the solar energy; and a coolant reservoir at the very top.”
The NCCL system is 1 meter in length and 1.2 meters in height, using 20 mm pipes and 1 mm thick copper frames. A 20 cm long, 40 cm high reservoir sits 75 cm above the PV module. Two 2 mm radiation filters flank the cooling channel, which varies in thickness at 1 mm, 2 mm, 5 mm, 10 mm, or 20 mm.
A PV panel made of tempered glass, an ethylene–vinyl acetate (EVA) layer, a silicon PV cell, another EVA layer, and a polyvinyl fluoride (PVF) layer is installed underneath. The panel’s efficiency, measured without cooling, stands at 14.98%. Researchers simulated the reservoir liquids using pure water or 5.3 parts per million (ppm) silver (Ag)-water nanofluid.
“This reservoir receives the heated, lower-density water from the solar panel below it, and this fluid motion pushes water from the reservoir to the heat sink section at the base of the loop, rejecting thermal energy from the loop to the body of water on which the PV system floats,” said the group.
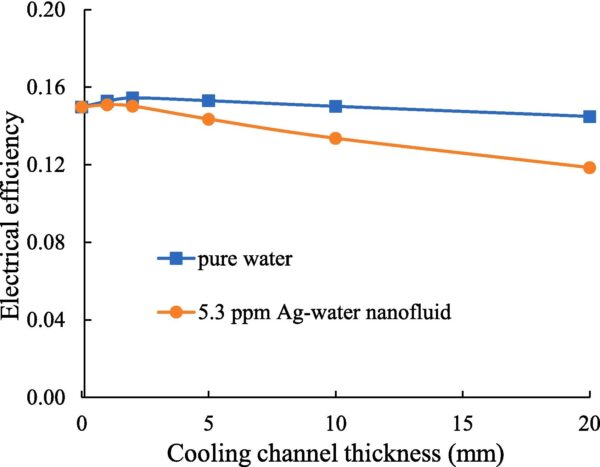
Image: University of Manchester, Solar Energy, CC BY 4.0
Scientists created a numerical model to describe the system, incorporating heat convection, conduction, radiation effects, and equations for fluid flow and heat transfer. They tested the model against experimental results, finding an average deviation of 8.3%. The model was further tested under 24-hour and eight-hour conditions in Bandung, Indonesia. The initial temperature was uniformly set at 298.15 K (25°C) across the solution domain, consistent with standard test conditions (STC).
“Variations in coolant channel thickness within the radiation filter above the photovoltaic module reveal that increasing channel thickness increases the buoyancy-driven coolant flow rate, which results in lower operating temperatures,” the researchers said. “As the cooling channel thickness increases, the coolant flow rate increment slows down. Increasing the coolant channel thickness also enhances the solar irradiance absorption, further lowering the photovoltaic cell temperatures, but at the expense of reducing the radiation energy available for electricity generation.”
Using pure water in the reservoir, the system achieved its highest efficiency of 15.45% with a 2 mm cooling channel thickness and its lowest efficiency of 14.48% at 20 mm. With 5.3 ppm Ag-water, efficiency peaked at 15.08% at 1 mm and dropped to 11.85% at 20 mm.
“Although the Ag-water nanofluid results in a lower temperature, the difference in efficiencies arises from pure water’s heightened transmissivity in the crystalline silicon photovoltaic cell’s useful wavelength range (325 nm to 1125 nm), whereas the Ag-water nanofluid absorbs more in the 340 nm – 510 nm,” said the academics. “Therefore, reducing the operating temperature alone does not guarantee higher efficiency, as the cooling fluid’s transmissivity within the useful wavelength range also plays a crucial role.”
They presented their results in “Efficiency improvement of floating photovoltaic panels with natural convection cooling loops: Multi-physics thermal modeling,” which was recently published in Solar Energy. In addition to scientists from the University of Manchester, the research group included academics from Politeknik Negeri Semarang in Indonesia, Guangdong Technion-Israel Institute of Technology in China, and Khalifa University in the United Arab Emirates.